Reversible and irreversible processes are concepts often discussed in thermodynamics. While they have key differences, there are some similarities as well. Let’s explore both:
Similarities:
- Change in State:
- Both reversible and irreversible processes involve a change in the state of a system. This change could be in terms of temperature, pressure, volume, or other thermodynamic properties.
- Energy Interaction:
- In both types of processes, there can be energy interactions with the surroundings. This energy exchange may occur as heat or work.
- System Equilibrium:
- Both processes involve the concept of equilibrium, though in different ways. Reversible processes are often described as being in equilibrium at every step, while irreversible processes may have some deviations from equilibrium.
- Thermodynamic Laws:
- Both types of processes adhere to the fundamental laws of thermodynamics, such as the conservation of energy and the second law of thermodynamics.
- Mathematical Descriptions:
- Both reversible and irreversible processes can be described using mathematical equations derived from thermodynamic principles. For example, the first law of thermodynamics applies to both types of processes.
Differences:
- Direction of Process:
- The most fundamental difference is the direction of the process. Reversible processes can proceed in either direction, while irreversible processes have a preferred direction.
- Path Dependence:
- Reversible processes are often characterized by the absence of path dependence. The final state of the system depends only on the initial and final states, not on the specific path taken. In contrast, irreversible processes can be path-dependent.
- Entropy Changes:
- Reversible processes are associated with minimal entropy changes, and the entropy changes are easily calculable. Irreversible processes, on the other hand, involve an increase in entropy.
- Idealization vs. Reality:
- Reversible processes are often idealizations that may not be achievable in the real world, as they require infinitely slow changes. Irreversible processes are more representative of actual physical processes.
- Efficiency:
- Reversible processes are idealized to be more efficient, while irreversible processes typically involve losses in efficiency, especially due to the increase in entropy.
Understanding these similarities and differences helps in grasping the core concepts of reversible and irreversible processes in thermodynamics.
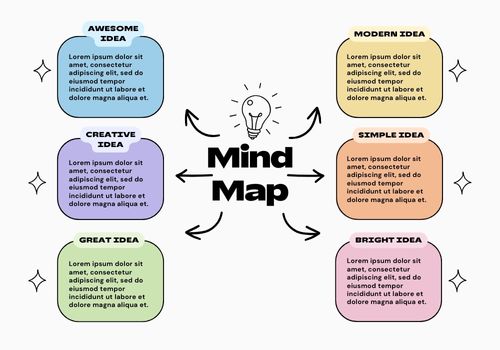
What is a reversible process?
A reversible process is a concept in thermodynamics that describes an idealized change in the state of a system that can be exactly reversed by an infinitesimally small change in some external condition. In simpler terms, a reversible process is one that can proceed in either direction with negligible deviation from equilibrium. It is an idealization and often serves as a theoretical benchmark for understanding the fundamental principles of thermodynamics.
Key characteristics of a reversible process include:
- Infinitesimal Steps: The process occurs in a series of infinitely small steps, each of which is in equilibrium. At each step, the system is in a state of thermodynamic equilibrium with its surroundings.
- Quasi-Static: The changes occur so slowly that the system remains in equilibrium throughout the entire process. This quasi-static nature ensures that the system continuously adjusts to the changing conditions without creating imbalances.
- No Irreversibilities: There are no irreversibilities or dissipative effects (such as friction, heat conduction, or fluid viscosity) within the system or its surroundings during a reversible process.
- Path Independence: The final state of the system depends only on the initial and final states, not on the specific path taken. This means that the process is path-independent and reversible, regardless of the specific sequence of intermediate states.
- Efficiency: Reversible processes are idealized as being more efficient than real-world processes. For example, a reversible heat engine operating between two temperature reservoirs would have the maximum possible efficiency according to the Carnot efficiency.
It’s important to note that truly reversible processes are often theoretical and rarely achievable in practice, as they would require infinite time and perfectly insulated systems. However, the concept of reversible processes is valuable for developing fundamental thermodynamic principles and understanding the theoretical limits of various thermodynamic processes.
What is a irreversible process?
An irreversible process is a thermodynamic process that cannot be undone or returned to its initial state by any practical means without some change in the system or its surroundings. In other words, once an irreversible process occurs, it leaves a lasting impact on the system and its environment. Irreversible processes are more common in real-world scenarios and are in contrast to the idealized reversible processes.
Key characteristics of irreversible processes include:
- Directionality: Irreversible processes have a preferred direction and occur spontaneously in one direction. Once they occur, it is not possible to exactly reverse the changes that took place.
- Irreversibilities: These processes involve irreversibilities or dissipative effects, such as friction, heat conduction, fluid viscosity, and other forms of energy dissipation. Irreversibilities lead to an increase in entropy within the system and its surroundings.
- Path Dependence: The final state of the system may depend on the specific path taken during the process. Unlike reversible processes, irreversible processes exhibit path dependence, meaning the final outcome is influenced by the particular sequence of intermediate states.
- Efficiency Loss: Irreversible processes are associated with losses in efficiency. For example, a real-world heat engine operating between two temperature reservoirs cannot achieve the efficiency of an idealized reversible Carnot engine.
- Spontaneous Nature: Irreversible processes often occur spontaneously, driven by natural forces or gradients. Examples include heat transfer from a hot object to a cold one, mixing of different substances, and many chemical reactions.
While reversible processes are often used as theoretical models to understand the principles of thermodynamics, irreversible processes are more representative of actual physical processes in the world. The second law of thermodynamics, which states that the entropy of an isolated system tends to increase over time, is closely related to the irreversibility of natural processes. The concept of irreversibility is crucial for understanding the limitations and efficiency of real-world thermodynamic systems.
What are the differences between reversible and irreversible process?
Reversible and irreversible processes in thermodynamics differ in several key aspects. Here are the main differences between the two:
- Direction of Process:
- Reversible Process: It can proceed in either direction without deviating from equilibrium. The process is ideally capable of being reversed by making infinitesimally small changes to external conditions.
- Irreversible Process: It has a preferred direction and occurs spontaneously in one direction. Once it occurs, it cannot be exactly undone, and there are lasting effects on the system and its surroundings.
- Infinitesimal Steps:
- Reversible Process: It occurs in a series of infinitely small, quasi-static steps, with the system in equilibrium at each step.
- Irreversible Process: It typically involves finite and often rapid changes, without maintaining equilibrium throughout the process.
- Path Dependence:
- Reversible Process: It is path-independent, meaning the final state depends only on the initial and final states and is not influenced by the specific path taken.
- Irreversible Process: It may exhibit path dependence, where the final state depends on the specific sequence of intermediate states during the process.
- Irreversibilities:
- Reversible Process: There are no irreversibilities or dissipative effects within the system or its surroundings.
- Irreversible Process: Irreversibilities, such as friction, heat conduction, and fluid viscosity, contribute to a net increase in entropy within the system and its surroundings.
- Efficiency:
- Reversible Process: It is idealized to be more efficient, with minimal energy losses.
- Irreversible Process: It is associated with losses in efficiency due to irreversibilities and an increase in entropy.
- Spontaneity:
- Reversible Process: It does not necessarily occur spontaneously; it can be driven in either direction if external conditions are adjusted accordingly.
- Irreversible Process: It often occurs spontaneously, driven by natural forces or gradients, without external intervention.
- Real-world Applicability:
- Reversible Process: Often theoretical and rarely achievable in practice, serving as an idealized benchmark for understanding fundamental thermodynamic principles.
- Irreversible Process: More representative of actual physical processes in the real world, reflecting the natural tendency of systems to evolve towards higher entropy states.
Understanding these differences is essential for grasping the conceptual distinctions between reversible and irreversible processes in the context of thermodynamics.
Table summarizing the similarities and differences between reversible and irreversible process
Here’s a table summarizing the key similarities and differences between reversible and irreversible processes:
Aspect | Reversible Process | Irreversible Process |
---|---|---|
Direction of Process | Can proceed in either direction without deviation from equilibrium. | Has a preferred direction and occurs spontaneously. |
Infinitesimal Steps | Occurs in a series of infinitely small, quasi-static steps, maintaining equilibrium. | Typically involves finite, often rapid changes without maintaining equilibrium. |
Path Dependence | Path-independent; final state depends only on initial and final states. | May exhibit path dependence; final state influenced by the specific path taken. |
Irreversibilities | No irreversibilities or dissipative effects within the system or surroundings. | Involves irreversibilities, leading to an increase in entropy. |
Efficiency | Idealized to be more efficient with minimal energy losses. | Associated with losses in efficiency due to irreversibilities. |
Spontaneity | Does not necessarily occur spontaneously; can be driven in either direction. | Often occurs spontaneously, driven by natural forces or gradients. |
Real-world Applicability | Often theoretical and rarely achievable in practice. | More representative of actual physical processes in the real world. |
Summary of Similarities Between reversible and irreversible processes
Here’s a summary of the similarities between reversible and irreversible processes:
- Change in State:
- Both reversible and irreversible processes involve a change in the state of a system, such as changes in temperature, pressure, volume, or other thermodynamic properties.
- Energy Interaction:
- In both types of processes, there can be energy interactions with the surroundings. This energy exchange may occur as heat or work.
- System Equilibrium:
- Both reversible and irreversible processes involve the concept of equilibrium, although the nature of equilibrium is different. Reversible processes maintain equilibrium at every step, while irreversible processes may have deviations from equilibrium.
- Thermodynamic Laws:
- Both types of processes adhere to the fundamental laws of thermodynamics, including the conservation of energy and the second law of thermodynamics.
- Mathematical Descriptions:
- Both reversible and irreversible processes can be described using mathematical equations derived from thermodynamic principles. For example, the first law of thermodynamics applies to both types of processes.
Understanding these similarities helps establish a foundation for comprehending the broader principles of thermodynamics and the commonalities in the behavior of systems undergoing reversible and irreversible processes.
Here are some frequently asked questions (FAQs) related to reversible and irreversible processes
Here are some frequently asked questions (FAQs) related to reversible and irreversible processes in thermodynamics:
- What is a reversible process?
- A reversible process is an idealized thermodynamic process that can be exactly undone by making infinitesimally small changes to external conditions. It occurs in a series of equilibrium states.
- What is an irreversible process?
- An irreversible process is a thermodynamic process that cannot be exactly undone, typically involving dissipative effects and leading to an increase in entropy.
- Why are reversible processes considered idealized?
- Reversible processes are considered idealized because they involve infinitely slow changes and lack irreversibility, making them theoretical benchmarks for understanding thermodynamic principles.
- How does entropy change in reversible and irreversible processes?
- In reversible processes, entropy changes are minimal or zero. In irreversible processes, there is an increase in entropy due to irreversibility.
- Can real-world processes be truly reversible?
- No, real-world processes often have irreversibility, and achieving true reversibility is impractical as it would require infinite time and perfectly insulated systems.
- What is the significance of reversible and irreversible processes in thermodynamics?
- Reversible processes help establish theoretical limits and principles in thermodynamics, while irreversible processes are more representative of actual physical processes, reflecting the tendency of systems to increase in entropy.
- How does the efficiency of reversible and irreversible processes compare?
- Reversible processes are idealized to be more efficient, while irreversible processes are associated with losses in efficiency due to irreversibility.
- Can irreversible processes be useful in practical applications?
- Yes, many practical processes, such as combustion engines and chemical reactions, are irreversible. Understanding and managing irreversibility are crucial for designing efficient systems.
- What role does the second law of thermodynamics play in reversible and irreversible processes?
- The second law of thermodynamics states that the entropy of an isolated system tends to increase over time. Irreversible processes align with this law, contributing to an overall increase in entropy.
- Are reversible processes achievable in the real world?
- Reversible processes are theoretical and often unattainable in real-world scenarios due to practical constraints, but they serve as important conceptual tools for understanding thermodynamics.
References
OpenStax and Paula Herrera-Siklody, (2016): Reversible and Irreversible Processes. Pressbooks
W. U. Schröder, Reversible and irreversible processes Rochester Univ.
J Zhao, (2022): A general framework to derive linear, decoupled and energy-stable schemes for reversible-irreversible thermodynamically consistent models. Elsevier
Leave a Reply